Using Effects-Directed Analysis to test for Endocrine-Active Chemicals
Effects-directed analysis (EDA) reverse-engineers environmental toxicity testing by measuring effects on cells first, and identifying the particular chemicals later. In this publication, we reviewed published information on EDA methods to improve future methods.
By Jessica Leet and Thea Edwards
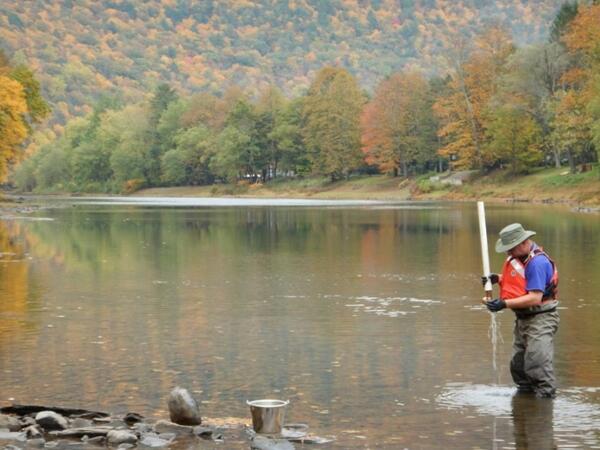
When people want to protect an environment, it is helpful to identify any chemical pollutants in that environment. Chemicals get particular attention if they potentially harm populations of fish or wildlife. In standard toxicology studies, scientists often test the effects of individual chemicals on fish or wildlife health. But, this approach is time-consuming and expensive, especially when you consider that the US Environmental Protection Agency has a list of over 83,000 chemicals in their Toxic Substances Inventory.
Additionally, in the environment, chemicals almost always occur in complex mixtures, not as isolated pollutants. Mixtures present several challenges. For example, methods are not always available to measure all the chemicals in the mixture. Even for chemicals that can be measured, they might occur at very low doses that still affect animal health, but are below the detection limits of the measurement method. Finally, chemicals in mixtures can behave differently than isolated chemicals and may be more toxic because they occur in a mixture.
Effects-directed analysis (EDA) is an alternative approach to identify chemicals that occur in complex mixtures. Instead of starting with a single chemical, this approach starts with observing how chemical mixtures in environmental samples affect cells grown in the laboratory. This type of biological test is called a “bioassay” and uses special cell lines that target a specific kind of toxicity related to expected or observed effects in fish or wildlife. EDAs can be used to test all kinds of samples like water or sediment (Figure 1) from polluted rivers, outflow from wastewater treatment plants, or soils from industrial sites. After the initial testing, scientists can go through additional steps to narrow down which chemical or chemicals in that sample are “active”, meaning they cause the observed effects in cells.
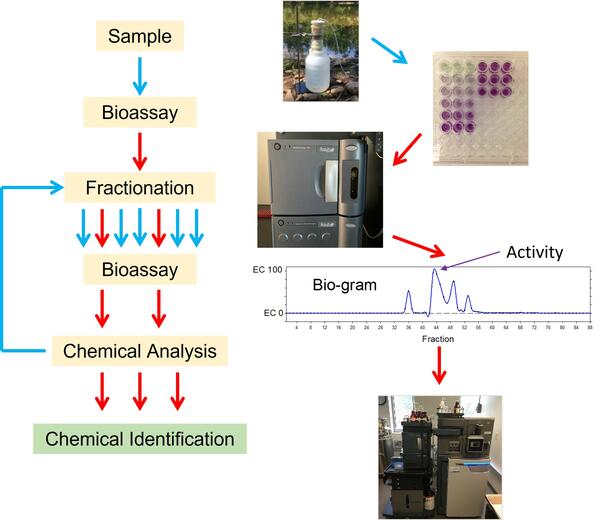
The basic structure of an EDA (Figure 2) starts with a field sample that is tested using a bioassay to determine if that sample has “activity”. If the sample has activity, the sample is broken into different chemical fractions. Fractions are separated based on their molecular mass, electrical charge, or other physical features that enable chemical separation. Then those fractions are tested again using the same bioassay to determine which fractions are active. If desired, active fractions can be further separated into more precise fractions to be tested. The specific chemicals present in the active fractions can then be identified using analytical chemistry methods.
Figure 2 shows the process of doing an EDA. In this way, scientists can identify which chemicals in a mixture are likely causing the adverse effects observed in fish or wildlife. This idea is then tested in the laboratory by exposing animals to the identified chemical(s) to determine if they do indeed cause the expected adverse effect.
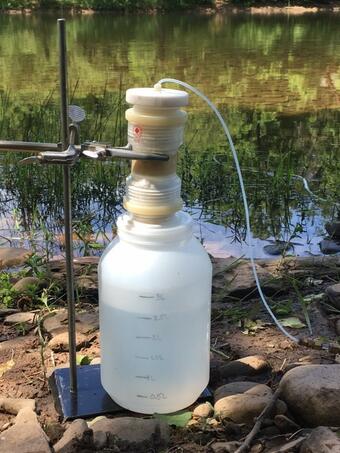
In our published paper, we did a critical review of the published information on EDAs and built a conceptual framework to guide future EDAs. For example, we built calculations to estimate how much water would need to be collected from the environment to be able to identify active chemicals (Figure 3). These calculations were based on examples of available bioassays, chemical methods used, and actual concentrations of chemicals measured in field samples.
From our review, we identified three key take‐away points to consider when using EDAs to assess toxicity of environmental samples: 1) the bioassay must be sensitive and specific enough to capture the biological effect of interest; 2) analytical chemistry is more sensitive than a bioassay most of the time; 3) current sampling techniques often cannot collect enough water for an EDA, particularly when the EDA is not very sensitive or the chemicals in the samples are not strongly active.
Based on our conceptual framework, we developed a guidance for choosing the bioassay, water volume, and sampling techniques for the most effective EDA. We hope this guidance provides a valuable tool for estimating the needs of sample collection, a crucial step in an EDA.
Return to Biochemistry and Physiology
Return to Endocrine Disruption
Fishing and Hunting Integrated Science Team
Effects-directed analysis (EDA) reverse-engineers environmental toxicity testing by measuring effects on cells first, and identifying the particular chemicals later. In this publication, we reviewed published information on EDA methods to improve future methods.
By Jessica Leet and Thea Edwards
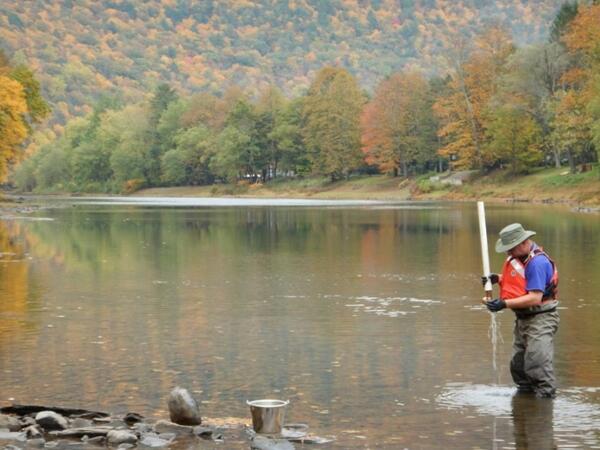
When people want to protect an environment, it is helpful to identify any chemical pollutants in that environment. Chemicals get particular attention if they potentially harm populations of fish or wildlife. In standard toxicology studies, scientists often test the effects of individual chemicals on fish or wildlife health. But, this approach is time-consuming and expensive, especially when you consider that the US Environmental Protection Agency has a list of over 83,000 chemicals in their Toxic Substances Inventory.
Additionally, in the environment, chemicals almost always occur in complex mixtures, not as isolated pollutants. Mixtures present several challenges. For example, methods are not always available to measure all the chemicals in the mixture. Even for chemicals that can be measured, they might occur at very low doses that still affect animal health, but are below the detection limits of the measurement method. Finally, chemicals in mixtures can behave differently than isolated chemicals and may be more toxic because they occur in a mixture.
Effects-directed analysis (EDA) is an alternative approach to identify chemicals that occur in complex mixtures. Instead of starting with a single chemical, this approach starts with observing how chemical mixtures in environmental samples affect cells grown in the laboratory. This type of biological test is called a “bioassay” and uses special cell lines that target a specific kind of toxicity related to expected or observed effects in fish or wildlife. EDAs can be used to test all kinds of samples like water or sediment (Figure 1) from polluted rivers, outflow from wastewater treatment plants, or soils from industrial sites. After the initial testing, scientists can go through additional steps to narrow down which chemical or chemicals in that sample are “active”, meaning they cause the observed effects in cells.
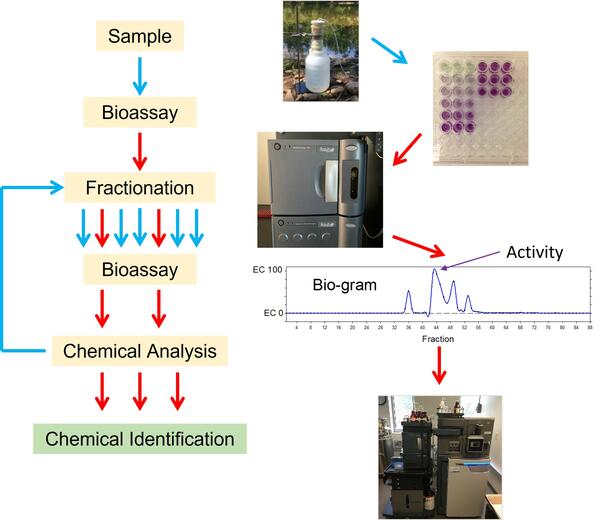
The basic structure of an EDA (Figure 2) starts with a field sample that is tested using a bioassay to determine if that sample has “activity”. If the sample has activity, the sample is broken into different chemical fractions. Fractions are separated based on their molecular mass, electrical charge, or other physical features that enable chemical separation. Then those fractions are tested again using the same bioassay to determine which fractions are active. If desired, active fractions can be further separated into more precise fractions to be tested. The specific chemicals present in the active fractions can then be identified using analytical chemistry methods.
Figure 2 shows the process of doing an EDA. In this way, scientists can identify which chemicals in a mixture are likely causing the adverse effects observed in fish or wildlife. This idea is then tested in the laboratory by exposing animals to the identified chemical(s) to determine if they do indeed cause the expected adverse effect.
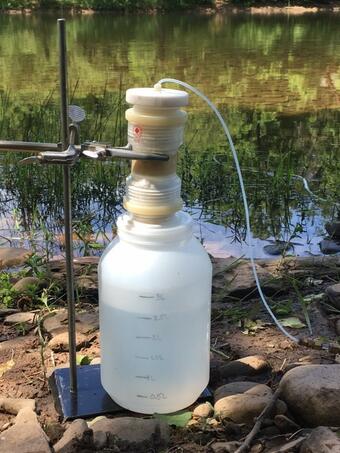
In our published paper, we did a critical review of the published information on EDAs and built a conceptual framework to guide future EDAs. For example, we built calculations to estimate how much water would need to be collected from the environment to be able to identify active chemicals (Figure 3). These calculations were based on examples of available bioassays, chemical methods used, and actual concentrations of chemicals measured in field samples.
From our review, we identified three key take‐away points to consider when using EDAs to assess toxicity of environmental samples: 1) the bioassay must be sensitive and specific enough to capture the biological effect of interest; 2) analytical chemistry is more sensitive than a bioassay most of the time; 3) current sampling techniques often cannot collect enough water for an EDA, particularly when the EDA is not very sensitive or the chemicals in the samples are not strongly active.
Based on our conceptual framework, we developed a guidance for choosing the bioassay, water volume, and sampling techniques for the most effective EDA. We hope this guidance provides a valuable tool for estimating the needs of sample collection, a crucial step in an EDA.
Return to Biochemistry and Physiology
Return to Endocrine Disruption