Preliminary Analysis of the Tsunami Generated by the June 23, 2001 Peru Earthquake
A preliminary analysis of the tsunami generated by the June 23, 2001 Peru earthquake is presented here.
Background
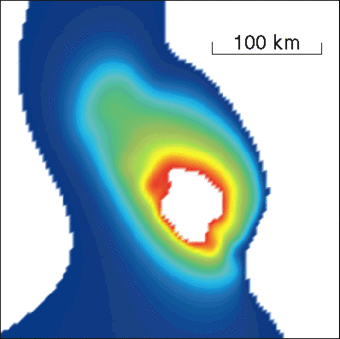
On June 23, 2001 a Mw=8.4 earthquake struck near the coast of southern Peru, 110 miles (175 km) west of Arequipa or about 370 miles (595 km) southeast of Lima. This great-sized earthquake resulted in many casualties, the majority of which were associated with the subsequent tsunami and landsliding in the Camaná-Chala region. The tsunami magnitude for this earthquake, calculated by Katsuyuki Abe of the Earthquake Research Institute, was Mt=8.2. For accurate information on the earthquake and its aftershocks, please see the USGS NEIC executive summary.
A model for tsunami generation and local propagation is presented here. For other models of far-field (trans-Pacific) propagation and observations of the tsunami, please see the NOAA/PMEL Center for Tsunami Research page.
Sedimentary deposits from the June 23, 2001 Peru tsunami were studied by a team of scientist from the US Geological Survey, University of California at Santa Cruz, University of Southern California, and government agencies and universities in Peru. View photographs of sedimentary deposits from the tsunami and field measurements of inundation distances and runup elevations.
Tsunami Generation
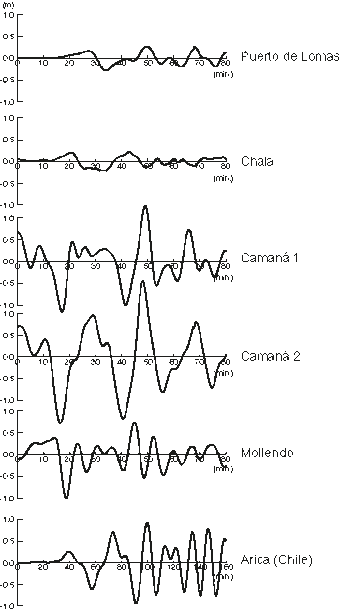
We calculate the tsunami generated by this earthquake using a seismic inversion of the rupture process determined by Kikuchi and Yamanaka at the Earthquake Research Institute, Univ. of Tokyo, Japan (from 7/12/01). Using the shallow-dipping nodal plane consistent with an interplate thrust earthquake along the Peru subduction zone, they determine the moment distribution over a rupture area 210 km long by 120 km wide (Mw=8.2). Details of their analysis are given on the Special Event Page for the Peru earthquake.
To calculate a slip distribution for the earthquake, we use a depth-dependent shear modulus function derived by Bilek and Lay (2000) from the source duration analysis of past events in this region. Coseismic vertical displacement predicted for this earthquake is shown here (color scale represents uplift—surrounding area is subsidence).
In performing these calculations, we note that not only does spatial variability of slip magnitude have a significant effect on the coseismic vertical displacement field (Geist and Dmowska, 1999), but variations in rake angle within the rupture zone also have a measurable effect on vertical surface displacement. The rupture process of this earthquake may be linked to initial arc-parallel stresses caused by the bend in the South American subduction zone in this region and/or subduction of the Nazca Ridge (Macharé and Ortlieb, 1992).
The Local Tsunami
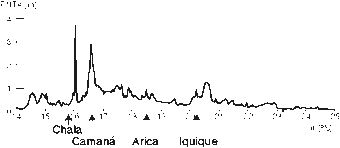
Coseismic vertical displacement provides the initial conditions for tsunami propagation. Because the rupture area extended landward of the coastline, only part of the coseismic deformation was transferred to generating a tsunami. Local propagation is calculated using a finite-difference approximation to the linear long-wave equations and the 2-minute Smith and Sandwell (1997) bathymetric data (Copyright). An 8 s time step was used in the propagation model. Reflection boundary conditions were imposed at the 50 m isobath. At this water depth, synthetic marigrams near offshore coastal communities are shown here. The actual wave response at the coast would have been modified by nearshore bathymetric changes and shoaling of the wave.
Please note that the time scale for the Arica, Chile synthetic marigram is compressed by a factor of 2 (0-160 min.) compared to the other stations (0-80 min.). For comparison to other modeling efforts that include tide gauge stations at greater distances, see the NOAA/PMEL Center for Tsunami Research page. As with other observed tsunamis, there are multiple waves that impact the coastline, arising from complex reflections and trapped modes. In most cases, the first/direct arrival is not the largest wave.
Tsunami runup can be roughly approximated by applying an amplification factor of 2 to the above PNTA values. Non-linear and overland flow models can more accurately simulate tsunami runup than the model used here. Maximum values of PNTA are located along the Chala-Camaná coastline of Peru, consistent with preliminary reports. In addition, there appears to be a secondary peak in PNTA south of Iquique, Chile. Shown below are snapshots from the tsunami propagation model (red-positive amplitude; blue-negative amplitude). Both the wavefield and topography are vertically exaggerated.
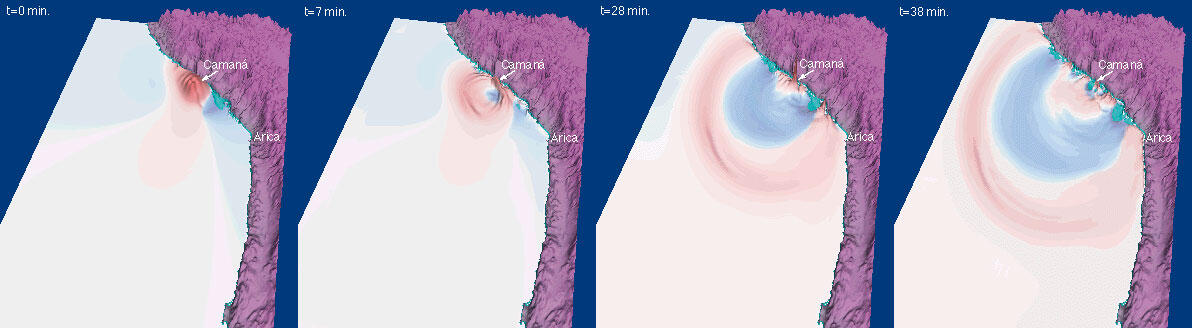
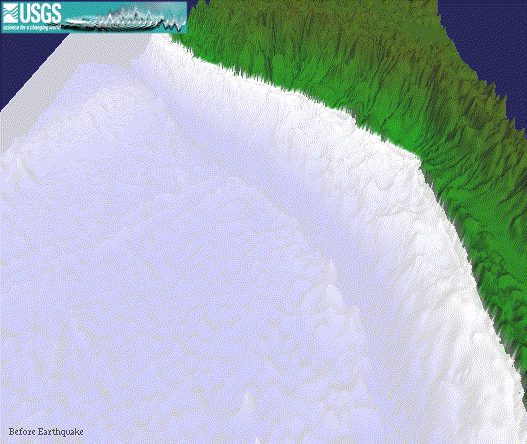
References Cited
Bilek, S. L., and T. Lay, 2000. Depth dependent rupture properties in Circum-Pacific subduction zones: In, GeoComplexity and the Physics of Earthquakes, J. B. Rundle, D. L. Turcotte, and W. Klein, eds., Geophys. Mon. 120, Am. Geophys. U., p. 165-186.
Geist, E.L., and R. Dmowska, 1999. Local tsunamis and distributed slip at the source: Pure Appl. Geophys., v. 154, p. 485-512.
Macharé, J., and L. Ortlieb, 1992. Plio-Quaternary vertical motions and the subduction of the Nazca Ridge, central coast of Peru: Tectonophysicics, v. 205., p. 97-108.
Smith, W. H. F., and D. T. Sandwell, 1997. Global Sea Floor Topography from Satellite Altimetry and Ship Depth Soundings: Science, v. 277, p. 1956-1962.
Yoshioka, S., M. Hashimoto, and K. Hirahara, 1989. Displacement fields due to the 1946 Nankaido earthquake in a laterally inhomogeneous structure with the subducting Philippine Sea plate—a three-dimensional finite element approach: Tectonophysics, v. 159, p. 121-136.
Web page produced by
Eric Geist (USGS, Pacific Coastal and Marine Science Center) with help from
Shoichi Yoshioka (Kyushu Univ., visiting Geological Survey of Canada)
Susan Bilek (UC Santa Cruz)
Kenji Hirata (JAMSTEC, visiting USGS)
We thank Prof. Kikuchi for supplying us with digital seismic inversion results.
Smith and Sandwell 1997 Copyright
Walter H. F. Smith and David T. Sandwell, Copyright 1997
Please copy and use these digital topography files for your applications. We are not responsible for errors in the depths. The depths have limited accuracy and should not be used for navigation.
The data contained in this digital file should not be used in any product sold for profit without the written consent of the authors.
Consent is not required for non-profit research use or for creating graphics for oral or poster presentations.
The appropriate reference for these data is: Smith, W. H. F. and Sandwell, D. T., Global Seafloor Topography from Satellite Altimetry and Ship Depth Soundings, submitted to Science, April 7, 1997.
This copyright text must be copied and placed in the same directory as the digital data file. In addition, the copyright must be attached to any derivative digital topography grids that are intended for re-distribution. Our objective is to have the data distributed as widely as possible, but not sold.
This copyright also applies to the Land Elevation data.
Below are other science projects associated with this project.
Tsunami and Earthquake Research
Preliminary Analysis of Sedimentary Deposits from the June 23, 2001 Peru Tsunami
A preliminary analysis of the tsunami generated by the June 23, 2001 Peru earthquake is presented here.
Background
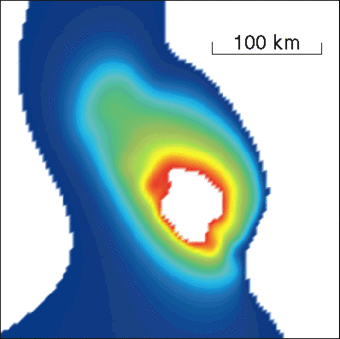
On June 23, 2001 a Mw=8.4 earthquake struck near the coast of southern Peru, 110 miles (175 km) west of Arequipa or about 370 miles (595 km) southeast of Lima. This great-sized earthquake resulted in many casualties, the majority of which were associated with the subsequent tsunami and landsliding in the Camaná-Chala region. The tsunami magnitude for this earthquake, calculated by Katsuyuki Abe of the Earthquake Research Institute, was Mt=8.2. For accurate information on the earthquake and its aftershocks, please see the USGS NEIC executive summary.
A model for tsunami generation and local propagation is presented here. For other models of far-field (trans-Pacific) propagation and observations of the tsunami, please see the NOAA/PMEL Center for Tsunami Research page.
Sedimentary deposits from the June 23, 2001 Peru tsunami were studied by a team of scientist from the US Geological Survey, University of California at Santa Cruz, University of Southern California, and government agencies and universities in Peru. View photographs of sedimentary deposits from the tsunami and field measurements of inundation distances and runup elevations.
Tsunami Generation
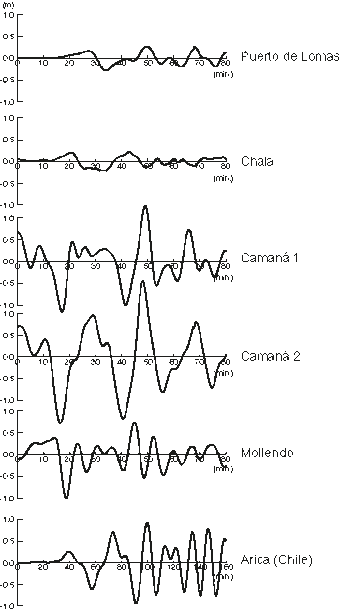
We calculate the tsunami generated by this earthquake using a seismic inversion of the rupture process determined by Kikuchi and Yamanaka at the Earthquake Research Institute, Univ. of Tokyo, Japan (from 7/12/01). Using the shallow-dipping nodal plane consistent with an interplate thrust earthquake along the Peru subduction zone, they determine the moment distribution over a rupture area 210 km long by 120 km wide (Mw=8.2). Details of their analysis are given on the Special Event Page for the Peru earthquake.
To calculate a slip distribution for the earthquake, we use a depth-dependent shear modulus function derived by Bilek and Lay (2000) from the source duration analysis of past events in this region. Coseismic vertical displacement predicted for this earthquake is shown here (color scale represents uplift—surrounding area is subsidence).
In performing these calculations, we note that not only does spatial variability of slip magnitude have a significant effect on the coseismic vertical displacement field (Geist and Dmowska, 1999), but variations in rake angle within the rupture zone also have a measurable effect on vertical surface displacement. The rupture process of this earthquake may be linked to initial arc-parallel stresses caused by the bend in the South American subduction zone in this region and/or subduction of the Nazca Ridge (Macharé and Ortlieb, 1992).
The Local Tsunami
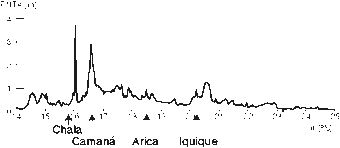
Coseismic vertical displacement provides the initial conditions for tsunami propagation. Because the rupture area extended landward of the coastline, only part of the coseismic deformation was transferred to generating a tsunami. Local propagation is calculated using a finite-difference approximation to the linear long-wave equations and the 2-minute Smith and Sandwell (1997) bathymetric data (Copyright). An 8 s time step was used in the propagation model. Reflection boundary conditions were imposed at the 50 m isobath. At this water depth, synthetic marigrams near offshore coastal communities are shown here. The actual wave response at the coast would have been modified by nearshore bathymetric changes and shoaling of the wave.
Please note that the time scale for the Arica, Chile synthetic marigram is compressed by a factor of 2 (0-160 min.) compared to the other stations (0-80 min.). For comparison to other modeling efforts that include tide gauge stations at greater distances, see the NOAA/PMEL Center for Tsunami Research page. As with other observed tsunamis, there are multiple waves that impact the coastline, arising from complex reflections and trapped modes. In most cases, the first/direct arrival is not the largest wave.
Tsunami runup can be roughly approximated by applying an amplification factor of 2 to the above PNTA values. Non-linear and overland flow models can more accurately simulate tsunami runup than the model used here. Maximum values of PNTA are located along the Chala-Camaná coastline of Peru, consistent with preliminary reports. In addition, there appears to be a secondary peak in PNTA south of Iquique, Chile. Shown below are snapshots from the tsunami propagation model (red-positive amplitude; blue-negative amplitude). Both the wavefield and topography are vertically exaggerated.
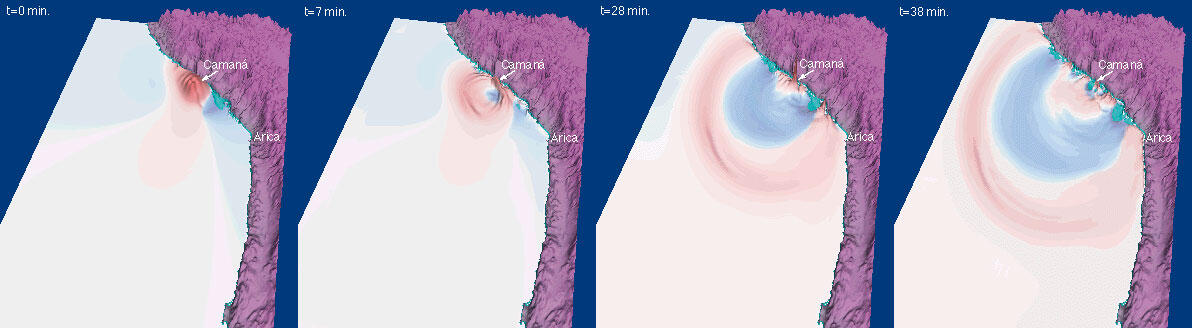
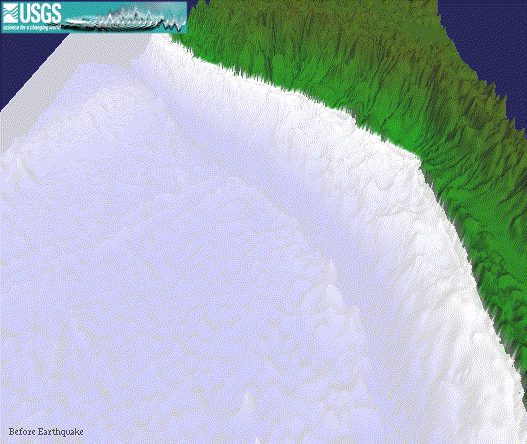
References Cited
Bilek, S. L., and T. Lay, 2000. Depth dependent rupture properties in Circum-Pacific subduction zones: In, GeoComplexity and the Physics of Earthquakes, J. B. Rundle, D. L. Turcotte, and W. Klein, eds., Geophys. Mon. 120, Am. Geophys. U., p. 165-186.
Geist, E.L., and R. Dmowska, 1999. Local tsunamis and distributed slip at the source: Pure Appl. Geophys., v. 154, p. 485-512.
Macharé, J., and L. Ortlieb, 1992. Plio-Quaternary vertical motions and the subduction of the Nazca Ridge, central coast of Peru: Tectonophysicics, v. 205., p. 97-108.
Smith, W. H. F., and D. T. Sandwell, 1997. Global Sea Floor Topography from Satellite Altimetry and Ship Depth Soundings: Science, v. 277, p. 1956-1962.
Yoshioka, S., M. Hashimoto, and K. Hirahara, 1989. Displacement fields due to the 1946 Nankaido earthquake in a laterally inhomogeneous structure with the subducting Philippine Sea plate—a three-dimensional finite element approach: Tectonophysics, v. 159, p. 121-136.
Web page produced by
Eric Geist (USGS, Pacific Coastal and Marine Science Center) with help from
Shoichi Yoshioka (Kyushu Univ., visiting Geological Survey of Canada)
Susan Bilek (UC Santa Cruz)
Kenji Hirata (JAMSTEC, visiting USGS)
We thank Prof. Kikuchi for supplying us with digital seismic inversion results.
Smith and Sandwell 1997 Copyright
Walter H. F. Smith and David T. Sandwell, Copyright 1997
Please copy and use these digital topography files for your applications. We are not responsible for errors in the depths. The depths have limited accuracy and should not be used for navigation.
The data contained in this digital file should not be used in any product sold for profit without the written consent of the authors.
Consent is not required for non-profit research use or for creating graphics for oral or poster presentations.
The appropriate reference for these data is: Smith, W. H. F. and Sandwell, D. T., Global Seafloor Topography from Satellite Altimetry and Ship Depth Soundings, submitted to Science, April 7, 1997.
This copyright text must be copied and placed in the same directory as the digital data file. In addition, the copyright must be attached to any derivative digital topography grids that are intended for re-distribution. Our objective is to have the data distributed as widely as possible, but not sold.
This copyright also applies to the Land Elevation data.
Below are other science projects associated with this project.