Earthquake Early Warning – Fine-Tuning for Best Alerts
Release Date: OCTOBER 8, 2019
The goal of an earthquake early warning (EEW) system is to provide an alert to people and automatic systems after an earthquake begins but before the shaking reaches their location. As the USGS and its partners are developing an EEW system, called ShakeAlert®, for the West Coast, the benefits, costs, capabilities, and limitations are being investigated. Two recent studies explored the timeliness and accuracy of alerts.
First, a little clarification about what EEW is and isn’t. EEW is not earthquake prediction. Earthquake prediction requires providing a date, a time, a location, and a magnitude before an earthquake occurs. Despite a great deal of scientific research, and many non-scientific claims, no one can yet predict earthquakes. EEW, on the other hand, is a warning that is sent after earthquake shaking has started. Since the transmission speed of the alert information is faster than the speed of the seismic waves, the alert can arrive in some places before the seismic waves arrive.
The two recent studies by USGS scientists and collaborators sought to determine 1) how quickly an alert can be generated, 2) how early a user might receive a warning before the strong shaking arrives, and 3) how accurately the shaking can be estimated. To explore how well the system could ideally perform, all three of these questions were answered by assuming a perfect EEW system; that is, one for which the current earthquake magnitude and location are known instantaneously and perfectly, and for which there is no lag time between the sent and received message. An important principle is that the locations farther away from where the earthquake begins will have more warning time, but the shaking won’t be as strong; locations close to the epicenter will have little or no warning time and will usually shake the strongest.
The basic seismological problem that you can’t get around is that a big earthquake looks just like a small earthquake in the first few seconds of rupture. If the earthquake rupture continues beyond a few seconds, you know it will be at least a moderate-size earthquake, and if it continues even beyond that, it will become a large earthquake. Large earthquakes shake a broader area and produce more intense shaking. As the earthquake is rupturing, the seismic waves are moving out from the rupture front, from the first seconds until the rupture finally ends, so it's a balancing act to figure out how long to wait with how much (or little) information before an alert is sent to beat the arriving seismic waves at your location.
To answer the first two enumerated questions above about alert timing, the first study (listed below) assumed that the shaking at any location could be estimated at any time during the rupture by measuring the equivalent magnitude of the event so far. As time marches on after the earthquake starts, it either keeps releasing more and more energy to become a large earthquake, or it stops rupturing and ends up being only a small or moderate-size earthquake. How quickly an alert can be issued, and how early before the shaking a user can receive the alert, depends on when you are willing to act. You might choose to act when shaking is estimated to be low, and receive many alerts that could end up being for small earthquakes with less intense shaking. If instead you waited to act until shaking is expected to be damaging, you would receive fewer overall alerts, but risk the chance of a too-late alert or not receiving an alert at all.
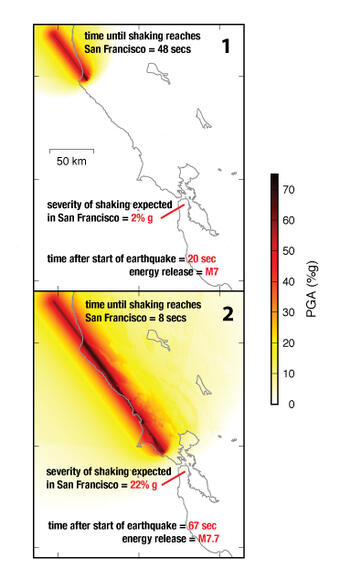
Consider the scenario of a magnitude 8 earthquake that starts on the northern San Andreas fault and ruptures south toward San Francisco (see image). This example is often considered to be a “best case scenario” for EEW. For reference, 20%g (where g is acceleration due to gravity) will produce a Modified Mercalli shaking intensity of MMI VII, which is the level at which we begin to expect moderate damage. Although this might seem like a reasonable threshold, if you lived in San Francisco with that alert setting, you would only get 8 seconds of warning before the strong shaking from this magnitude 8 earthquake arrived. And that’s assuming there are no delays or latencies in the system. If instead you were alerted when shaking was predicted to be only 2% g (corresponding to MMI IV, which is below the threshold for damage), you would get potentially 48 seconds of warning. At this low ground motion threshold, you would have more time to take protective actions in case the earthquake grew to be even larger than it was at the time the alert was issued (M 7).
For answering the third question about alert accuracy, the second study (listed below) assumed that the final earthquake location, magnitude, and rupture length could be determined instantaneously when the earthquake starts. Under these unrealizable conditions, the only unknown is what the shaking will be at any location, where the user is waiting for an alert. The predicted level of shaking could be determined quickly, and the alert would be sent if this level exceeded what the user has set as their minimum shaking level at that location for an alert. Even under such ideal conditions, the uncertainty in the shaking prediction is large enough that unless a user wants to receive more alerts than they really need to take action for, they may get an alert too late or not at all (a missed alert). Even if given more time, it is difficult to predict shaking intensities from earthquakes of a given magnitude, due to the variability in shaking due to variations in the earthquake rupture process, complex geologic structures the waves pass through and local site conditions.
There are many other sources of uncertainty and latency in a real-world EEW system that provide additional motivation for alerting at somewhat lower levels of anticipated ground motion than what can cause damage, and can make it even more likely that alerts will arrive after the shaking starts (which is anticipated to be the case for many users located close to the epicenter), and even missed alerts. Both studies concluded that even without added real-world issues, the optimal warning strategy is a “better safe than sorry” approach where users are warned if there is even a small chance that the earthquake will produce damaging ground motion. Although alerting at lower ground motion thresholds will result in users being alerted more often and for non-damaging shaking, they will be less likely to miss an alert for an infrequent larger earthquake with more intense shaking.
- written by Lisa Wald, U.S. Geological Survey, October 11, 2019
For More Information
- Minson, Sarah E., Meier Men-Andrin, Annemarie S. Baltay, Thomas C. Hanks, Elizabeth S. Cochran, 2018, The limits of earthquake early warning: Timeliness of ground motion estimates, Science Advances, v.4, n.3, DOI: 10.1126/sciadv.aaq0504.
- Minson, Sarah E., Annemarie S. Baltay, Elizabeth S. Cochran, Thomas C. Hanks, Morgan T. Page, Sara K. McBride, Kevin R. Milner, Men-Andriin Meier, 2019, The Limits of Earthquake Early Warning: Accuracy and Best Alerting Strategy, Scientific Reports 9, art.2478.
- ShakeAlert
- Earthquake Early Warning Research
The Scientists Behind the Science
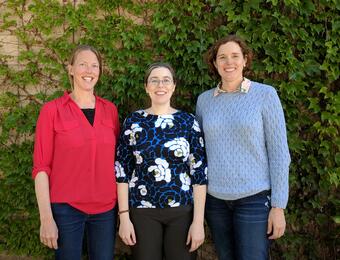
Sarah Minson is a research geophysicist with the USGS's Earthquake Science Center. Her research interests include using probabilistic inference for seismological problems such as determining the physics of earthquake ruptures, and estimating the slip distribution and predicting the ground motion from earthquakes in real-time for earthquake early warning. When not working on science, she follows instructions from her cat.
Annemarie Baltay is an observational seismologist with the USGS in Menlo Park who researches earthquake ground motions. She is interested in how earthquake source physics and earth materials/structure control ground motions measured at distances from an earthquake, and how we use observations of ground motion to infer information about the physics of the earth. When not thinking about ground shaking, she likes to bike and hike with her family.
Elizabeth Cochran is a seismologist with the USGS where she has worked since 2011. She uses records of earthquake waves to determine what faults look like and the details of how earthquakes break along those faults. Elizabeth is particularly interested in how earthquakes are triggered, both naturally and by human activities. She has also developed new tools to record earthquake using sensors that can be run by volunteers, including at schools and museums. When she is not chasing earthquakes, Elizabeth enjoys spending time with her husband, Moh, and their children, Corinna and Simon.
Release Date: OCTOBER 8, 2019
The goal of an earthquake early warning (EEW) system is to provide an alert to people and automatic systems after an earthquake begins but before the shaking reaches their location. As the USGS and its partners are developing an EEW system, called ShakeAlert®, for the West Coast, the benefits, costs, capabilities, and limitations are being investigated. Two recent studies explored the timeliness and accuracy of alerts.
First, a little clarification about what EEW is and isn’t. EEW is not earthquake prediction. Earthquake prediction requires providing a date, a time, a location, and a magnitude before an earthquake occurs. Despite a great deal of scientific research, and many non-scientific claims, no one can yet predict earthquakes. EEW, on the other hand, is a warning that is sent after earthquake shaking has started. Since the transmission speed of the alert information is faster than the speed of the seismic waves, the alert can arrive in some places before the seismic waves arrive.
The two recent studies by USGS scientists and collaborators sought to determine 1) how quickly an alert can be generated, 2) how early a user might receive a warning before the strong shaking arrives, and 3) how accurately the shaking can be estimated. To explore how well the system could ideally perform, all three of these questions were answered by assuming a perfect EEW system; that is, one for which the current earthquake magnitude and location are known instantaneously and perfectly, and for which there is no lag time between the sent and received message. An important principle is that the locations farther away from where the earthquake begins will have more warning time, but the shaking won’t be as strong; locations close to the epicenter will have little or no warning time and will usually shake the strongest.
The basic seismological problem that you can’t get around is that a big earthquake looks just like a small earthquake in the first few seconds of rupture. If the earthquake rupture continues beyond a few seconds, you know it will be at least a moderate-size earthquake, and if it continues even beyond that, it will become a large earthquake. Large earthquakes shake a broader area and produce more intense shaking. As the earthquake is rupturing, the seismic waves are moving out from the rupture front, from the first seconds until the rupture finally ends, so it's a balancing act to figure out how long to wait with how much (or little) information before an alert is sent to beat the arriving seismic waves at your location.
To answer the first two enumerated questions above about alert timing, the first study (listed below) assumed that the shaking at any location could be estimated at any time during the rupture by measuring the equivalent magnitude of the event so far. As time marches on after the earthquake starts, it either keeps releasing more and more energy to become a large earthquake, or it stops rupturing and ends up being only a small or moderate-size earthquake. How quickly an alert can be issued, and how early before the shaking a user can receive the alert, depends on when you are willing to act. You might choose to act when shaking is estimated to be low, and receive many alerts that could end up being for small earthquakes with less intense shaking. If instead you waited to act until shaking is expected to be damaging, you would receive fewer overall alerts, but risk the chance of a too-late alert or not receiving an alert at all.
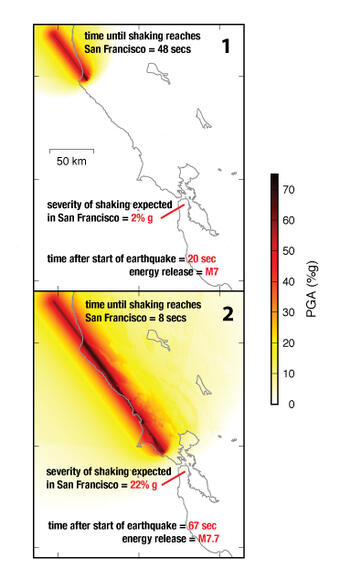
Consider the scenario of a magnitude 8 earthquake that starts on the northern San Andreas fault and ruptures south toward San Francisco (see image). This example is often considered to be a “best case scenario” for EEW. For reference, 20%g (where g is acceleration due to gravity) will produce a Modified Mercalli shaking intensity of MMI VII, which is the level at which we begin to expect moderate damage. Although this might seem like a reasonable threshold, if you lived in San Francisco with that alert setting, you would only get 8 seconds of warning before the strong shaking from this magnitude 8 earthquake arrived. And that’s assuming there are no delays or latencies in the system. If instead you were alerted when shaking was predicted to be only 2% g (corresponding to MMI IV, which is below the threshold for damage), you would get potentially 48 seconds of warning. At this low ground motion threshold, you would have more time to take protective actions in case the earthquake grew to be even larger than it was at the time the alert was issued (M 7).
For answering the third question about alert accuracy, the second study (listed below) assumed that the final earthquake location, magnitude, and rupture length could be determined instantaneously when the earthquake starts. Under these unrealizable conditions, the only unknown is what the shaking will be at any location, where the user is waiting for an alert. The predicted level of shaking could be determined quickly, and the alert would be sent if this level exceeded what the user has set as their minimum shaking level at that location for an alert. Even under such ideal conditions, the uncertainty in the shaking prediction is large enough that unless a user wants to receive more alerts than they really need to take action for, they may get an alert too late or not at all (a missed alert). Even if given more time, it is difficult to predict shaking intensities from earthquakes of a given magnitude, due to the variability in shaking due to variations in the earthquake rupture process, complex geologic structures the waves pass through and local site conditions.
There are many other sources of uncertainty and latency in a real-world EEW system that provide additional motivation for alerting at somewhat lower levels of anticipated ground motion than what can cause damage, and can make it even more likely that alerts will arrive after the shaking starts (which is anticipated to be the case for many users located close to the epicenter), and even missed alerts. Both studies concluded that even without added real-world issues, the optimal warning strategy is a “better safe than sorry” approach where users are warned if there is even a small chance that the earthquake will produce damaging ground motion. Although alerting at lower ground motion thresholds will result in users being alerted more often and for non-damaging shaking, they will be less likely to miss an alert for an infrequent larger earthquake with more intense shaking.
- written by Lisa Wald, U.S. Geological Survey, October 11, 2019
For More Information
- Minson, Sarah E., Meier Men-Andrin, Annemarie S. Baltay, Thomas C. Hanks, Elizabeth S. Cochran, 2018, The limits of earthquake early warning: Timeliness of ground motion estimates, Science Advances, v.4, n.3, DOI: 10.1126/sciadv.aaq0504.
- Minson, Sarah E., Annemarie S. Baltay, Elizabeth S. Cochran, Thomas C. Hanks, Morgan T. Page, Sara K. McBride, Kevin R. Milner, Men-Andriin Meier, 2019, The Limits of Earthquake Early Warning: Accuracy and Best Alerting Strategy, Scientific Reports 9, art.2478.
- ShakeAlert
- Earthquake Early Warning Research
The Scientists Behind the Science
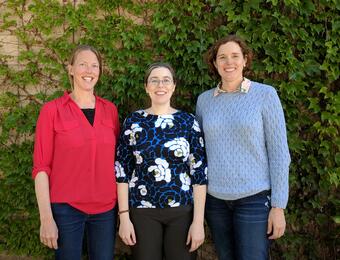
Sarah Minson is a research geophysicist with the USGS's Earthquake Science Center. Her research interests include using probabilistic inference for seismological problems such as determining the physics of earthquake ruptures, and estimating the slip distribution and predicting the ground motion from earthquakes in real-time for earthquake early warning. When not working on science, she follows instructions from her cat.
Annemarie Baltay is an observational seismologist with the USGS in Menlo Park who researches earthquake ground motions. She is interested in how earthquake source physics and earth materials/structure control ground motions measured at distances from an earthquake, and how we use observations of ground motion to infer information about the physics of the earth. When not thinking about ground shaking, she likes to bike and hike with her family.
Elizabeth Cochran is a seismologist with the USGS where she has worked since 2011. She uses records of earthquake waves to determine what faults look like and the details of how earthquakes break along those faults. Elizabeth is particularly interested in how earthquakes are triggered, both naturally and by human activities. She has also developed new tools to record earthquake using sensors that can be run by volunteers, including at schools and museums. When she is not chasing earthquakes, Elizabeth enjoys spending time with her husband, Moh, and their children, Corinna and Simon.