North River Air pilot Taj Shoemaker getting ready for takeoff in Prince William Sound.
Nearshore ecosystems include many resources that are of high ecological, recreational, subsistence, and economic value. They also are subject to influences from a wide variety of natural and human-caused perturbations, which can originate in terrestrial or oceanic environments. Our research is designed to evaluate sources of variation in the nearshore and how they influence resources of high conservation interest.
Return to Ecosystems >> Marine Ecosystems
Sea Otter Population Assessment
With the exception of 13 small remnant populations, sea otters were extirpated from their historic range in the north Pacific Ocean during the 18th and 19th centuries as a result of the commercial harvest for their fur. During most of the 20th century, through protection and reintroduction, sea otter populations generally increased in abundance and distribution such that most of their range in Alaska, with the exception of southeast Alaska, was occupied by 2000. Although population abundance data are incomplete, there is evidence of increasing, stable and declining sea otter populations in different areas within their range. The factors that ultimately regulate sea otter population abundance are not completely understood, but can include predation, human harvest, food limitation, disease and catastrophic events such as oil spill. There is good evidence that the recent declines in sea otters in SW Alaska are related to killer whale predation and the Exxon Valdez oil spill reduced the size of the western Prince William Sound population in 1989. Human harvest of sea otters can adversely affect sea otter abundance, evidenced by the commercial fur trade leading to near extirpation. Because sea otters occupy relatively small home ranges and do not migrate, sustainable harvest requires management at appropriate spatial scales. Recently, harvest of sea otters for subsistence have been increasing, although effects of the harvest at current levels on population trend are unknown. Because sea otter populations occur over vast and remote areas and may display divergent trends in abundance over relatively small spatial scales, determining population status and trends can be challenging.
Methods to assess sea otter population status and trends are important to evaluate the recovery of populations and the potential effects of human perturbations (e.g., harvest, contaminants, and habitat modifications) on populations. This information is important to resource managers in identifying potential conflicts, identifying mechanisms of change, and improving the ability to detect and respond to change from human induced sources.
Objectives of our sea otter population assessment studies include: 1) develop and test methods to identify the degree of population structuring among north Pacific sea otter populations, 2) develop and test techniques to accurately and precisely estimate the status of sea otter populations, 3) develop and test methods to identify cause(s) of change in the status and numeric trends of sea otter populations, 4) develop and test methods to determine the role of density dependent processes in affecting change in sea otter populations, and 5) evaluate the effects of population reductions and translocations on sea otter genetic variability.
Role of Sea Otters in Structuring Nearshore Communities
Sea otters provide one of the best documented examples of top-down forcing effects on the structure and function of nearshore marine ecosystems in the North Pacific Ocean. Much of our knowledge of the role of sea otters as a source of community variation resulted from the spatial and temporal pattern of sea otter population recovery since their near extirpation about 100 years ago. During most of the early 20th century sea otters were absent from large portions of their habitat in the north Pacific. During the absence of sea otters, many of their prey populations responded to reduced predation through increased densities and sizes. Since the middle of the 20th century sea otter populations have been recovering previous habitats, due to natural dispersal and translocations. Following the recovery of sea otters, scientists have continued to provide descriptions of nearshore marine communities and have been able to contrast those communities before and after the sea otters return. At least three distinct approaches have proven valuable in understanding the effects of sea otters. One is contrasting communities over time, before and after recolonization by sea otters. This approach, in concert with appropriate controls, provides an experimentally rigorous and powerful study design allowing inference to the cause of the observed changes in experimental areas. Another approach consists of contrasting different areas at the same time, those with, and those without the experimental treatment (in this case, sea otters). A third approach entails experimentally manipulating community attributes and observing community response, usually in both treatment and control areas. All these opportunities currently present themselves at various locations throughout the sea otters’ range.
One area of recent reoccupation is Glacier Bay in Southeast Alaska, where sea otters were absent until as recently as 1994, but currently number > 4000 individuals. We are using this situation in Glacier Bay as a laboratory to experimentally evaluate the role of sea otter in structuring coastal marine communities in a predominately soft sediment habitat. It is predictable that the density and sizes of preferred sea otter prey such as crabs, clams, and urchins will decline in response to otter predation. This will result in fewer opportunities for human harvest, but will also result in ecosystem level changes, as abundance and sizes of prey for other predators, such as octopus, sea stars, fishes, birds and mammals are modified. Sea otters will also modify benthic habitats through excavation of sediments required to extract burrowing infauna such as clams. Effects of sediment disturbance by foraging sea otters are not understood. As the recolonization by sea otters continues, it is also likely that dramatic changes will occur in the species composition, abundance and size class composition of many components of the nearshore marine ecosystem. Many of the changes will occur as a direct result of predation by sea otters; other changes will result from indirect or cascading effects of sea otter foraging, such as increasing kelp production and modified prey availability for other nearshore predators.
Effects of the Exxon Valdez Oil Spill
Sea otters were severely impacted by the 1989 Exxon Valdez oil spill. Estimates of acute spill related mortality range from about 1,000 to 5,500 in the first months after the spill. Scientists with the Alaska Science Center were among the first responders to the 1989 spill and continue work today to document the process of recovery form this spill and to better understand the effects future contamination events on sea otters and the nearshore ecosystems they occupy.
One of the factors limiting our ability to clearly understand and document the spill effects was a lack of accurate estimates of sea otter abundance. This was true for nearly all species in the Gulf of Alaska and remains an impediment in assessing injury from such catastrophes across most landscapes today. Initial research efforts following the spill focused on damage assessment, including developing methods to accurately estimate the abundance of affected populations and studies of reproduction and survival.
Large scale ecosystem level studies of nearshore species and habitats most affected by the spill completed in 1999, found evidence of long-term spill effects among nearshore species dependent on a nearshore food web where benthic invertebrates transfer primary production to upper level consumers such as sea otters and sea ducks. Biochemical and gene techniques suggested that lingering oil may have contributed to a protracted recovery period for nearshore species. Subsequently, surveys of beaches where oil was deposited nearly a decade earlier found unanticipated volumes of oil sequestered in nearly 20 acres of widely distributed soft sediment intertidal beaches in Prince William Sound.
Our most recent surveys of sea otter abundance indicate significant progress toward recovery, when we consider the entire spill affected area in the Sound. By 2009 our estimate of sea otter abundance in the western Sound was nearly 2,000 animals more than our first post spill estimate in 1993 of about 2,000 individuals. However, when we look only at those areas that were most severely affected by the spill, where sea otter mortality approached 90% and where much of the lingering oil has been located, evidence of recovery remains incomplete. Our most recent research, based on the diving behavior of sea otters in the intertidal and published oil encounter rates, indicates that all sea otters in those heavily oiled areas are likely to encounter Exxon Valdez oil at least annually and some as often as weekly. Long term continuation of studies investigating mortality from the annual collections of beach cast sea otter carcasses implicates elevated mortality as the factor most likely contributing to delayed recovery, and suggests that chronic mortality after the spill may meet or exceed the acute mortality experienced after the spill.
Lingering Oil Studies
Sea otters were severely impacted by the 1989 Exxon Valdez oil spill. Estimates of acute spill related mortality range from about 1,000 to 5,500 in the first months after the spill. Scientists with the Alaska Science Center were among the first responders to the 1989 spill and continue work today to document the process of recovery form this spill and to better understand the effects future contamination events on sea otters and the nearshore ecosystems they occupy.
One of the factors limiting our ability to clearly understand and document the spill effects was a lack of accurate estimates of sea otter abundance. This was true for nearly all species in the Gulf of Alaska and remains an impediment in assessing injury from such catastrophes across most landscapes today. Initial research efforts following the spill focused on damage assessment, including developing methods to accurately estimate the abundance of affected populations and studies of reproduction and survival.
Large scale ecosystem level studies of nearshore species and habitats most affected by the spill completed in 1999, found evidence of long-term spill effects among nearshore species dependent on a nearshore food web where benthic invertebrates transfer primary production to upper level consumers such as sea otters and sea ducks. Biochemical and gene techniques suggested that lingering oil may have contributed to a protracted recovery period for nearshore species. Subsequently, surveys of beaches where oil was deposited nearly a decade earlier found unanticipated volumes of oil sequestered in nearly 20 acres of widely distributed soft sediment intertidal beaches in Prince William Sound.
Our most recent surveys of sea otter abundance indicate significant progress toward recovery, when we consider the entire spill affected area in the Sound. By 2009 our estimate of sea otter abundance in the western Sound was nearly 2,000 animals more than our first post spill estimate in 1993 of about 2,000 individuals. However, when we look only at those areas that were most severely affected by the spill, where sea otter mortality approached 90% and where much of the lingering oil has been located, evidence of recovery remains incomplete. Our most recent research, based on the diving behavior of sea otters in the intertidal and published oil encounter rates, indicates that all sea otters in those heavily oiled areas are likely to encounter Exxon Valdez oil at least annually and some as often as weekly. Long term continuation of studies investigating mortality from the annual collections of beach cast sea otter carcasses implicates elevated mortality as the factor most likely contributing to delayed recovery, and suggests that chronic mortality after the spill may meet or exceed the acute mortality experienced after the spill.
Long-term Monitoring
The Alaska Science Center, and in preceding Department of Interior agencies, has been engaged in monitoring various sea otter populations for more than 50 years, since Karl Kenyon’s seminal work in the Aleutian Islands. As sea otter populations have recovered from the fur trade and translocations contributed to expanding populations, the task of sea otter monitoring has become increasingly difficult simply because of the vast and remote nature of sea otter habitat. Moreover, it has become increasingly evident that monitoring of single species, while perhaps necessary for management purposes, often provides little insight as to why changes in abundance occur over time. As a result we have been engaged in the development, design and testing of monitoring protocols for nearshore habitats and species, including sea otters, that might best be described as “ecosystem” or “food web” based monitoring.
The nearshore is considered an important component of the Gulf of Alaska ecosystem, including the region affected by the Exxon Valdez oil spill, because it provides:
- A variety of unique habitats for resident organisms (e.g. sea otters, harbor seals, shorebirds, seabirds, nearshore fishes, kelps, seagrasses, clams, mussels, and sea stars).
- Nursery grounds for marine animals from other habitats (e.g. crabs, salmon, herring, and seabirds).
- Feeding grounds for important consumers, including killer whales, harbor seals, sea otters, sea lions, sea ducks, shore birds and many fish and shellfish.
- A source of animals important to commercial and subsistence harvests (e.g. marine mammals, fishes, crabs, mussels, clams, chitons, and octopus).
- An important site of recreational activities including fishing, boating, camping, and nature viewing.
- A source of primary production for export to adjacent habitats (primarily by kelps, other seaweeds, and eelgrass), as well as a recipient for primary (phytoplankton) and secondary production (zooplankton) transported from offshore systems..
- An important triple interface between air, land and sea that provides linkages for transfer of water, nutrients, and species between watersheds and offshore habitats.
The underlying assumption in our monitoring design is that change will occur, and that careful consideration of what to monitor, may eventually provide insight as to why observed change occurred. In the nearshore ecosystem we work in, primary productivity is provided by at least two independent sources, the micro-algae, or phytoplankton, that occurs near the sea surface and may be transported inshore via currents. The second, and sometimes major contributor to total primary production is through the kelps and sea grasses that are conspicuous features of the nearshore zone. These combined sources of carbon fuel a diverse community of invertebrates, such as mussels, clams, snails, crustaceans, and urchins, that ultimately transfer their energy to various higher trophic level invertebrates and vertebrates, such as fishes, birds (shore birds, sea ducks and others) and mammals (primarily sea otters). Through careful selection of species and processes (growth, survival and diet) we expect to gain a better understanding of the interaction between various trophic levels that will allow us to potentially assign cause to some of the change we expect to see over time.
As part of the planning efforts of the Exxon Valdez Trustee Council for a long-term science program, in 2001 we were tasked to develop a science and monitoring program for the nearshore ecosystem in the Gulf of Alaska. Through a process of workshops and consultations we developed the Nearshore Restoration and Ecosystem Monitoring program (N-REM, Dean and Bodkin 2006). Coincident with our planning efforts for the Exxon Valdez Trustte Council, the National Park Service was implementing a strategy known as “vital signs monitoring” to develop scientifically sound information on the status and long-term trends of park ecosystems and to determine how well current management practices are sustaining those ecosystems. Subsequently, Park managers from the Southwest Alaska Network (SWAN) recognized that the program we designed for the Exxon Valdez Trustee Council fit their Vital Signs needs and a new partnership was established to implement long term monitoring in the nearshore marine habitat of the SWAN parks.
SWAN consists of five Alaskan park units (Aniakchak National Monument and Preserve, Alagnak National Wild River, Katmai National Park and Preserve, Kenai Fjords National Park, and Lake Clark National Park and Preserve). Collectively these units comprise 9.4 million acres or 11.6 percent of the total land area managed by the National Park Service. Network parks encompass climatic conditions, geologic features, near pristine ecosystems, natural biodiversity, freshwater, and marine resources equaled few places in North America. This network of relatively untouched wilderness parks is a unique resource and offers unparalleled opportunities to study and monitor ecological systems minimally affected by humans. In recognition of this, the SWAN monitoring framework emphasizes (i) establishing reference conditions representing the current status of park, monument, and preserve ecosystems; and (ii) detecting ecological change through time. In 2008, The Exxon Valdez Trustee Council adopted and implemented our nearshore monitoring design in Prince William Sound, extending the SWAN nearshore program from the Gulf of Alaska into Prince William Sound and Kachemak Bay in Cook Inlet. The Gulf of Alaska nearshore monitoring program now consists of four primary sites, including Prince William Sound, Kenai Fjords National Park, Kachemak Bay and Katmai National Park.
Below are data or web applications associated with this project.
Sea Otter Aerial Survey Data from lower Cook Inlet and the outer Kenai Penisula, Alaska, 2002
Sea Otter Aerial Survey Data from Western Prince William Sound, Alaska, 2022
Glacier Bay National Park and Preserve Sea Otter Forage Data, 1993-2019
North Pacific Wintering Barrow's Goldeneye Body Mass, Morphology, and Prey Sizes 1996-2015
Rocky Intertidal Data from Prince William Sound, Katmai National Park and Preserve, and Kenai Fjords National Park
Intertidal Temperature Data from Kachemak Bay, Prince William Sound, Katmai National Park and Preserve, and Kenai Fjords National Park
Sea Otter Spraint Data from Kachemak Bay, Katmai National Park and Preserve, Kenai Fjords National Park and Prince William Sound
Intertidal Mussel (Mytilus) Data from Prince William Sound, Katmai National Park and Preserve, and Kenai Fjords National Park
Intertidal and Subtidal Sea Otter Prey Sampling in Mixed Sediment Habitat in Glacier Bay National Park and Preserve, Alaska, 1998 to 2011
Sea Otter Aerial Survey Data from Lower Cook Inlet, Alaska, 2017
Bioenergetics and Morphology of Mussels (Mytilus trossulus) in Kenai Fjords National Park
Sea Otter Aerial Survey Data from the outer Kenai Peninsula, Alaska, 2019
Below are multimedia items associated with this project.
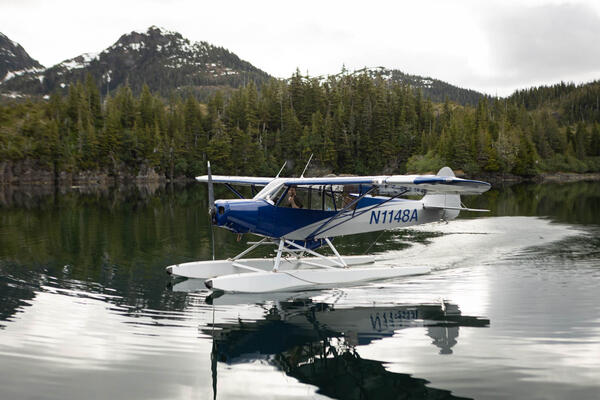
North River Air pilot Taj Shoemaker getting ready for takeoff in Prince William Sound.
A resting sea otter with eyes closed. Photo taken in the Homer harbor in Alaska. Sea otters provide one of the best documented examples of top-down forcing effects on the structure and function of nearshore marine ecosystems in the North Pacific Ocean
A resting sea otter with eyes closed. Photo taken in the Homer harbor in Alaska. Sea otters provide one of the best documented examples of top-down forcing effects on the structure and function of nearshore marine ecosystems in the North Pacific Ocean
A resting sea otter in the Homer harbor, Alaska. Photo was taken in 2011. Sea otters provide one of the best documented examples of top-down forcing effects on the structure and function of nearshore marine ecosystems in the North Pacific Ocean.
A resting sea otter in the Homer harbor, Alaska. Photo was taken in 2011. Sea otters provide one of the best documented examples of top-down forcing effects on the structure and function of nearshore marine ecosystems in the North Pacific Ocean.
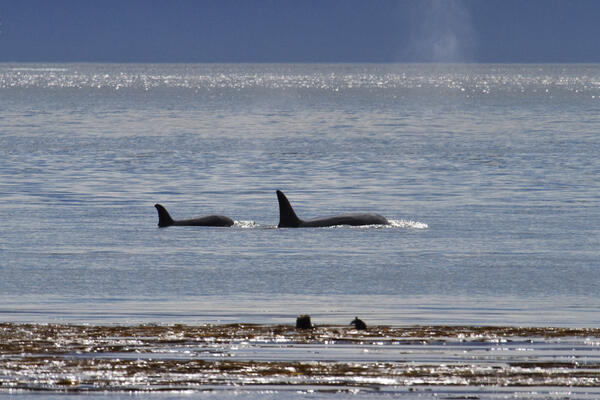
A sea otter resting in kelp as two orcas swim past. Photo taken in Glacier Bay at Point Carolus, Alaska
A sea otter resting in kelp as two orcas swim past. Photo taken in Glacier Bay at Point Carolus, Alaska
A female sea otter resting in the morning light in Cook Inlet Alaska.
A female sea otter resting in the morning light in Cook Inlet Alaska.
A young sea otter foraging for small invertebrates off an emergent rock covered with seaweed, snails, and barnacles. Photo taken in Prince William Sound, Alaska.
A young sea otter foraging for small invertebrates off an emergent rock covered with seaweed, snails, and barnacles. Photo taken in Prince William Sound, Alaska.
A sea otter mom nursing her pup. Photo taken in Prince William Sound, Alaska. A new born sea otter needs to stay with its mother for six months to learn how to survive on its own.
A sea otter mom nursing her pup. Photo taken in Prince William Sound, Alaska. A new born sea otter needs to stay with its mother for six months to learn how to survive on its own.
A juvenile female sea otter eating small green urchins in the intertidal. Photo taken in Glacier Bay near Geikie Inlet, Alaska.
A juvenile female sea otter eating small green urchins in the intertidal. Photo taken in Glacier Bay near Geikie Inlet, Alaska.
A sea otter eating a basket star in Glacier Bay near Bartlett Cove, Alaska
A sea otter eating a basket star in Glacier Bay near Bartlett Cove, Alaska
Below are publications associated with this project.
Understanding sea otter population change in southeast Alaska
Informing management of recovering predators and their prey with ecological diffusion models
Changes in abiotic drivers of green sea urchin demographics following the loss of a keystone predator
Gene expression and wildlife health: Varied interpretations based on perspective
Northern Sea Otter (Enhydra lutris kenyoni) population abundance and distribution across the southeast Alaska stock, summer 2022
Brown bear–sea otter interactions along the Katmai coast: Terrestrial and nearshore communities linked by predation
Where land and sea meet: Brown bears and sea otters
Abundance and distribution of sea otters (Enhydra lutris) in the southcentral Alaska stock, 2014, 2017, and 2019
Diffusion modeling reveals effects of multiple release sites and human activity on a recolonizing apex predator
Translocations maintain genetic diversity and increase connectivity in sea otters, Enhydra lutris
Sea otter predator avoidance behavior
Biological correlates of sea urchin recruitment in kelp forest and urchin barren habitats
Below are news stories associated with this project.
Nearshore ecosystems include many resources that are of high ecological, recreational, subsistence, and economic value. They also are subject to influences from a wide variety of natural and human-caused perturbations, which can originate in terrestrial or oceanic environments. Our research is designed to evaluate sources of variation in the nearshore and how they influence resources of high conservation interest.
Return to Ecosystems >> Marine Ecosystems
Sea Otter Population Assessment
With the exception of 13 small remnant populations, sea otters were extirpated from their historic range in the north Pacific Ocean during the 18th and 19th centuries as a result of the commercial harvest for their fur. During most of the 20th century, through protection and reintroduction, sea otter populations generally increased in abundance and distribution such that most of their range in Alaska, with the exception of southeast Alaska, was occupied by 2000. Although population abundance data are incomplete, there is evidence of increasing, stable and declining sea otter populations in different areas within their range. The factors that ultimately regulate sea otter population abundance are not completely understood, but can include predation, human harvest, food limitation, disease and catastrophic events such as oil spill. There is good evidence that the recent declines in sea otters in SW Alaska are related to killer whale predation and the Exxon Valdez oil spill reduced the size of the western Prince William Sound population in 1989. Human harvest of sea otters can adversely affect sea otter abundance, evidenced by the commercial fur trade leading to near extirpation. Because sea otters occupy relatively small home ranges and do not migrate, sustainable harvest requires management at appropriate spatial scales. Recently, harvest of sea otters for subsistence have been increasing, although effects of the harvest at current levels on population trend are unknown. Because sea otter populations occur over vast and remote areas and may display divergent trends in abundance over relatively small spatial scales, determining population status and trends can be challenging.
Methods to assess sea otter population status and trends are important to evaluate the recovery of populations and the potential effects of human perturbations (e.g., harvest, contaminants, and habitat modifications) on populations. This information is important to resource managers in identifying potential conflicts, identifying mechanisms of change, and improving the ability to detect and respond to change from human induced sources.
Objectives of our sea otter population assessment studies include: 1) develop and test methods to identify the degree of population structuring among north Pacific sea otter populations, 2) develop and test techniques to accurately and precisely estimate the status of sea otter populations, 3) develop and test methods to identify cause(s) of change in the status and numeric trends of sea otter populations, 4) develop and test methods to determine the role of density dependent processes in affecting change in sea otter populations, and 5) evaluate the effects of population reductions and translocations on sea otter genetic variability.
Role of Sea Otters in Structuring Nearshore Communities
Sea otters provide one of the best documented examples of top-down forcing effects on the structure and function of nearshore marine ecosystems in the North Pacific Ocean. Much of our knowledge of the role of sea otters as a source of community variation resulted from the spatial and temporal pattern of sea otter population recovery since their near extirpation about 100 years ago. During most of the early 20th century sea otters were absent from large portions of their habitat in the north Pacific. During the absence of sea otters, many of their prey populations responded to reduced predation through increased densities and sizes. Since the middle of the 20th century sea otter populations have been recovering previous habitats, due to natural dispersal and translocations. Following the recovery of sea otters, scientists have continued to provide descriptions of nearshore marine communities and have been able to contrast those communities before and after the sea otters return. At least three distinct approaches have proven valuable in understanding the effects of sea otters. One is contrasting communities over time, before and after recolonization by sea otters. This approach, in concert with appropriate controls, provides an experimentally rigorous and powerful study design allowing inference to the cause of the observed changes in experimental areas. Another approach consists of contrasting different areas at the same time, those with, and those without the experimental treatment (in this case, sea otters). A third approach entails experimentally manipulating community attributes and observing community response, usually in both treatment and control areas. All these opportunities currently present themselves at various locations throughout the sea otters’ range.
One area of recent reoccupation is Glacier Bay in Southeast Alaska, where sea otters were absent until as recently as 1994, but currently number > 4000 individuals. We are using this situation in Glacier Bay as a laboratory to experimentally evaluate the role of sea otter in structuring coastal marine communities in a predominately soft sediment habitat. It is predictable that the density and sizes of preferred sea otter prey such as crabs, clams, and urchins will decline in response to otter predation. This will result in fewer opportunities for human harvest, but will also result in ecosystem level changes, as abundance and sizes of prey for other predators, such as octopus, sea stars, fishes, birds and mammals are modified. Sea otters will also modify benthic habitats through excavation of sediments required to extract burrowing infauna such as clams. Effects of sediment disturbance by foraging sea otters are not understood. As the recolonization by sea otters continues, it is also likely that dramatic changes will occur in the species composition, abundance and size class composition of many components of the nearshore marine ecosystem. Many of the changes will occur as a direct result of predation by sea otters; other changes will result from indirect or cascading effects of sea otter foraging, such as increasing kelp production and modified prey availability for other nearshore predators.
Effects of the Exxon Valdez Oil Spill
Sea otters were severely impacted by the 1989 Exxon Valdez oil spill. Estimates of acute spill related mortality range from about 1,000 to 5,500 in the first months after the spill. Scientists with the Alaska Science Center were among the first responders to the 1989 spill and continue work today to document the process of recovery form this spill and to better understand the effects future contamination events on sea otters and the nearshore ecosystems they occupy.
One of the factors limiting our ability to clearly understand and document the spill effects was a lack of accurate estimates of sea otter abundance. This was true for nearly all species in the Gulf of Alaska and remains an impediment in assessing injury from such catastrophes across most landscapes today. Initial research efforts following the spill focused on damage assessment, including developing methods to accurately estimate the abundance of affected populations and studies of reproduction and survival.
Large scale ecosystem level studies of nearshore species and habitats most affected by the spill completed in 1999, found evidence of long-term spill effects among nearshore species dependent on a nearshore food web where benthic invertebrates transfer primary production to upper level consumers such as sea otters and sea ducks. Biochemical and gene techniques suggested that lingering oil may have contributed to a protracted recovery period for nearshore species. Subsequently, surveys of beaches where oil was deposited nearly a decade earlier found unanticipated volumes of oil sequestered in nearly 20 acres of widely distributed soft sediment intertidal beaches in Prince William Sound.
Our most recent surveys of sea otter abundance indicate significant progress toward recovery, when we consider the entire spill affected area in the Sound. By 2009 our estimate of sea otter abundance in the western Sound was nearly 2,000 animals more than our first post spill estimate in 1993 of about 2,000 individuals. However, when we look only at those areas that were most severely affected by the spill, where sea otter mortality approached 90% and where much of the lingering oil has been located, evidence of recovery remains incomplete. Our most recent research, based on the diving behavior of sea otters in the intertidal and published oil encounter rates, indicates that all sea otters in those heavily oiled areas are likely to encounter Exxon Valdez oil at least annually and some as often as weekly. Long term continuation of studies investigating mortality from the annual collections of beach cast sea otter carcasses implicates elevated mortality as the factor most likely contributing to delayed recovery, and suggests that chronic mortality after the spill may meet or exceed the acute mortality experienced after the spill.
Lingering Oil Studies
Sea otters were severely impacted by the 1989 Exxon Valdez oil spill. Estimates of acute spill related mortality range from about 1,000 to 5,500 in the first months after the spill. Scientists with the Alaska Science Center were among the first responders to the 1989 spill and continue work today to document the process of recovery form this spill and to better understand the effects future contamination events on sea otters and the nearshore ecosystems they occupy.
One of the factors limiting our ability to clearly understand and document the spill effects was a lack of accurate estimates of sea otter abundance. This was true for nearly all species in the Gulf of Alaska and remains an impediment in assessing injury from such catastrophes across most landscapes today. Initial research efforts following the spill focused on damage assessment, including developing methods to accurately estimate the abundance of affected populations and studies of reproduction and survival.
Large scale ecosystem level studies of nearshore species and habitats most affected by the spill completed in 1999, found evidence of long-term spill effects among nearshore species dependent on a nearshore food web where benthic invertebrates transfer primary production to upper level consumers such as sea otters and sea ducks. Biochemical and gene techniques suggested that lingering oil may have contributed to a protracted recovery period for nearshore species. Subsequently, surveys of beaches where oil was deposited nearly a decade earlier found unanticipated volumes of oil sequestered in nearly 20 acres of widely distributed soft sediment intertidal beaches in Prince William Sound.
Our most recent surveys of sea otter abundance indicate significant progress toward recovery, when we consider the entire spill affected area in the Sound. By 2009 our estimate of sea otter abundance in the western Sound was nearly 2,000 animals more than our first post spill estimate in 1993 of about 2,000 individuals. However, when we look only at those areas that were most severely affected by the spill, where sea otter mortality approached 90% and where much of the lingering oil has been located, evidence of recovery remains incomplete. Our most recent research, based on the diving behavior of sea otters in the intertidal and published oil encounter rates, indicates that all sea otters in those heavily oiled areas are likely to encounter Exxon Valdez oil at least annually and some as often as weekly. Long term continuation of studies investigating mortality from the annual collections of beach cast sea otter carcasses implicates elevated mortality as the factor most likely contributing to delayed recovery, and suggests that chronic mortality after the spill may meet or exceed the acute mortality experienced after the spill.
Long-term Monitoring
The Alaska Science Center, and in preceding Department of Interior agencies, has been engaged in monitoring various sea otter populations for more than 50 years, since Karl Kenyon’s seminal work in the Aleutian Islands. As sea otter populations have recovered from the fur trade and translocations contributed to expanding populations, the task of sea otter monitoring has become increasingly difficult simply because of the vast and remote nature of sea otter habitat. Moreover, it has become increasingly evident that monitoring of single species, while perhaps necessary for management purposes, often provides little insight as to why changes in abundance occur over time. As a result we have been engaged in the development, design and testing of monitoring protocols for nearshore habitats and species, including sea otters, that might best be described as “ecosystem” or “food web” based monitoring.
The nearshore is considered an important component of the Gulf of Alaska ecosystem, including the region affected by the Exxon Valdez oil spill, because it provides:
- A variety of unique habitats for resident organisms (e.g. sea otters, harbor seals, shorebirds, seabirds, nearshore fishes, kelps, seagrasses, clams, mussels, and sea stars).
- Nursery grounds for marine animals from other habitats (e.g. crabs, salmon, herring, and seabirds).
- Feeding grounds for important consumers, including killer whales, harbor seals, sea otters, sea lions, sea ducks, shore birds and many fish and shellfish.
- A source of animals important to commercial and subsistence harvests (e.g. marine mammals, fishes, crabs, mussels, clams, chitons, and octopus).
- An important site of recreational activities including fishing, boating, camping, and nature viewing.
- A source of primary production for export to adjacent habitats (primarily by kelps, other seaweeds, and eelgrass), as well as a recipient for primary (phytoplankton) and secondary production (zooplankton) transported from offshore systems..
- An important triple interface between air, land and sea that provides linkages for transfer of water, nutrients, and species between watersheds and offshore habitats.
The underlying assumption in our monitoring design is that change will occur, and that careful consideration of what to monitor, may eventually provide insight as to why observed change occurred. In the nearshore ecosystem we work in, primary productivity is provided by at least two independent sources, the micro-algae, or phytoplankton, that occurs near the sea surface and may be transported inshore via currents. The second, and sometimes major contributor to total primary production is through the kelps and sea grasses that are conspicuous features of the nearshore zone. These combined sources of carbon fuel a diverse community of invertebrates, such as mussels, clams, snails, crustaceans, and urchins, that ultimately transfer their energy to various higher trophic level invertebrates and vertebrates, such as fishes, birds (shore birds, sea ducks and others) and mammals (primarily sea otters). Through careful selection of species and processes (growth, survival and diet) we expect to gain a better understanding of the interaction between various trophic levels that will allow us to potentially assign cause to some of the change we expect to see over time.
As part of the planning efforts of the Exxon Valdez Trustee Council for a long-term science program, in 2001 we were tasked to develop a science and monitoring program for the nearshore ecosystem in the Gulf of Alaska. Through a process of workshops and consultations we developed the Nearshore Restoration and Ecosystem Monitoring program (N-REM, Dean and Bodkin 2006). Coincident with our planning efforts for the Exxon Valdez Trustte Council, the National Park Service was implementing a strategy known as “vital signs monitoring” to develop scientifically sound information on the status and long-term trends of park ecosystems and to determine how well current management practices are sustaining those ecosystems. Subsequently, Park managers from the Southwest Alaska Network (SWAN) recognized that the program we designed for the Exxon Valdez Trustee Council fit their Vital Signs needs and a new partnership was established to implement long term monitoring in the nearshore marine habitat of the SWAN parks.
SWAN consists of five Alaskan park units (Aniakchak National Monument and Preserve, Alagnak National Wild River, Katmai National Park and Preserve, Kenai Fjords National Park, and Lake Clark National Park and Preserve). Collectively these units comprise 9.4 million acres or 11.6 percent of the total land area managed by the National Park Service. Network parks encompass climatic conditions, geologic features, near pristine ecosystems, natural biodiversity, freshwater, and marine resources equaled few places in North America. This network of relatively untouched wilderness parks is a unique resource and offers unparalleled opportunities to study and monitor ecological systems minimally affected by humans. In recognition of this, the SWAN monitoring framework emphasizes (i) establishing reference conditions representing the current status of park, monument, and preserve ecosystems; and (ii) detecting ecological change through time. In 2008, The Exxon Valdez Trustee Council adopted and implemented our nearshore monitoring design in Prince William Sound, extending the SWAN nearshore program from the Gulf of Alaska into Prince William Sound and Kachemak Bay in Cook Inlet. The Gulf of Alaska nearshore monitoring program now consists of four primary sites, including Prince William Sound, Kenai Fjords National Park, Kachemak Bay and Katmai National Park.
Below are data or web applications associated with this project.
Sea Otter Aerial Survey Data from lower Cook Inlet and the outer Kenai Penisula, Alaska, 2002
Sea Otter Aerial Survey Data from Western Prince William Sound, Alaska, 2022
Glacier Bay National Park and Preserve Sea Otter Forage Data, 1993-2019
North Pacific Wintering Barrow's Goldeneye Body Mass, Morphology, and Prey Sizes 1996-2015
Rocky Intertidal Data from Prince William Sound, Katmai National Park and Preserve, and Kenai Fjords National Park
Intertidal Temperature Data from Kachemak Bay, Prince William Sound, Katmai National Park and Preserve, and Kenai Fjords National Park
Sea Otter Spraint Data from Kachemak Bay, Katmai National Park and Preserve, Kenai Fjords National Park and Prince William Sound
Intertidal Mussel (Mytilus) Data from Prince William Sound, Katmai National Park and Preserve, and Kenai Fjords National Park
Intertidal and Subtidal Sea Otter Prey Sampling in Mixed Sediment Habitat in Glacier Bay National Park and Preserve, Alaska, 1998 to 2011
Sea Otter Aerial Survey Data from Lower Cook Inlet, Alaska, 2017
Bioenergetics and Morphology of Mussels (Mytilus trossulus) in Kenai Fjords National Park
Sea Otter Aerial Survey Data from the outer Kenai Peninsula, Alaska, 2019
Below are multimedia items associated with this project.
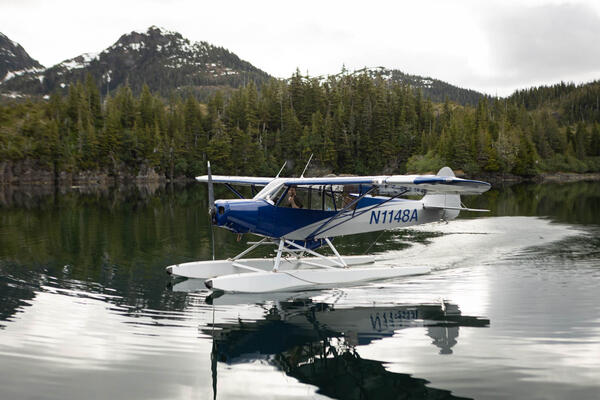
North River Air pilot Taj Shoemaker getting ready for takeoff in Prince William Sound.
North River Air pilot Taj Shoemaker getting ready for takeoff in Prince William Sound.
A resting sea otter with eyes closed. Photo taken in the Homer harbor in Alaska. Sea otters provide one of the best documented examples of top-down forcing effects on the structure and function of nearshore marine ecosystems in the North Pacific Ocean
A resting sea otter with eyes closed. Photo taken in the Homer harbor in Alaska. Sea otters provide one of the best documented examples of top-down forcing effects on the structure and function of nearshore marine ecosystems in the North Pacific Ocean
A resting sea otter in the Homer harbor, Alaska. Photo was taken in 2011. Sea otters provide one of the best documented examples of top-down forcing effects on the structure and function of nearshore marine ecosystems in the North Pacific Ocean.
A resting sea otter in the Homer harbor, Alaska. Photo was taken in 2011. Sea otters provide one of the best documented examples of top-down forcing effects on the structure and function of nearshore marine ecosystems in the North Pacific Ocean.
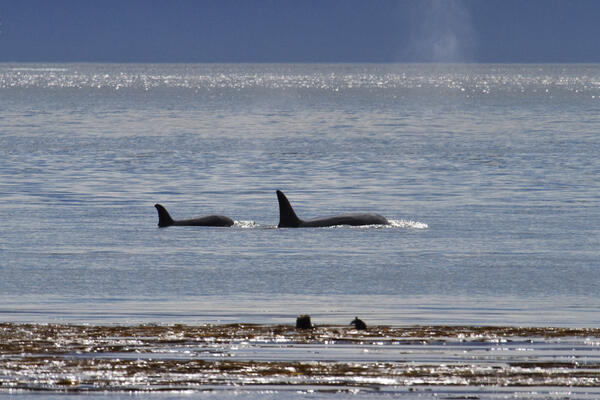
A sea otter resting in kelp as two orcas swim past. Photo taken in Glacier Bay at Point Carolus, Alaska
A sea otter resting in kelp as two orcas swim past. Photo taken in Glacier Bay at Point Carolus, Alaska
A female sea otter resting in the morning light in Cook Inlet Alaska.
A female sea otter resting in the morning light in Cook Inlet Alaska.
A young sea otter foraging for small invertebrates off an emergent rock covered with seaweed, snails, and barnacles. Photo taken in Prince William Sound, Alaska.
A young sea otter foraging for small invertebrates off an emergent rock covered with seaweed, snails, and barnacles. Photo taken in Prince William Sound, Alaska.
A sea otter mom nursing her pup. Photo taken in Prince William Sound, Alaska. A new born sea otter needs to stay with its mother for six months to learn how to survive on its own.
A sea otter mom nursing her pup. Photo taken in Prince William Sound, Alaska. A new born sea otter needs to stay with its mother for six months to learn how to survive on its own.
A juvenile female sea otter eating small green urchins in the intertidal. Photo taken in Glacier Bay near Geikie Inlet, Alaska.
A juvenile female sea otter eating small green urchins in the intertidal. Photo taken in Glacier Bay near Geikie Inlet, Alaska.
A sea otter eating a basket star in Glacier Bay near Bartlett Cove, Alaska
A sea otter eating a basket star in Glacier Bay near Bartlett Cove, Alaska
Below are publications associated with this project.
Understanding sea otter population change in southeast Alaska
Informing management of recovering predators and their prey with ecological diffusion models
Changes in abiotic drivers of green sea urchin demographics following the loss of a keystone predator
Gene expression and wildlife health: Varied interpretations based on perspective
Northern Sea Otter (Enhydra lutris kenyoni) population abundance and distribution across the southeast Alaska stock, summer 2022
Brown bear–sea otter interactions along the Katmai coast: Terrestrial and nearshore communities linked by predation
Where land and sea meet: Brown bears and sea otters
Abundance and distribution of sea otters (Enhydra lutris) in the southcentral Alaska stock, 2014, 2017, and 2019
Diffusion modeling reveals effects of multiple release sites and human activity on a recolonizing apex predator
Translocations maintain genetic diversity and increase connectivity in sea otters, Enhydra lutris
Sea otter predator avoidance behavior
Biological correlates of sea urchin recruitment in kelp forest and urchin barren habitats
Below are news stories associated with this project.